- TOP
- Cutting-edge Research and Social Contribution
- Cutting-edge Research
- Pursuing super-energy-saving photonics employing highly diverse organic materials
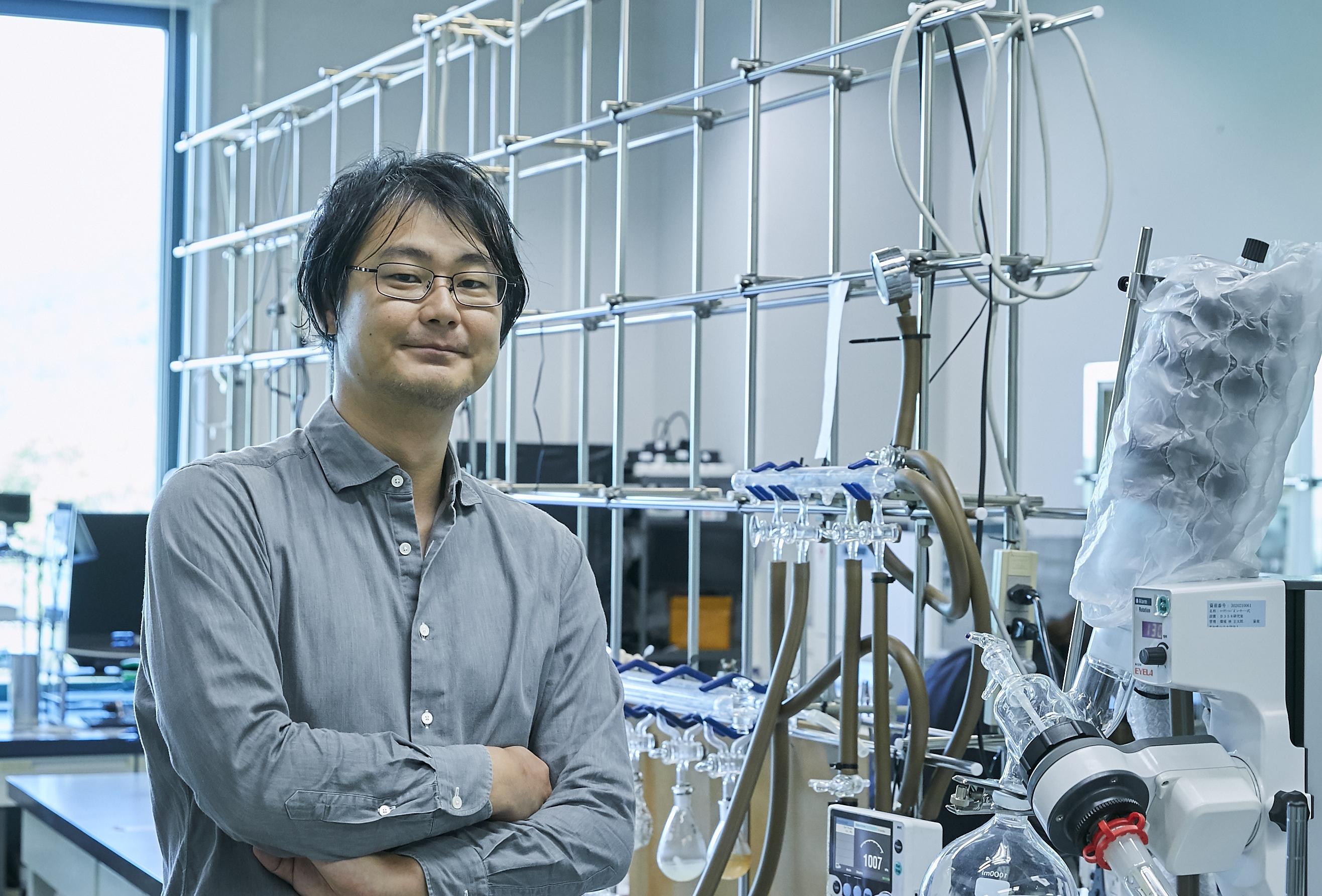
Pursuing super-energy-saving photonics employing highly diverse organic materials
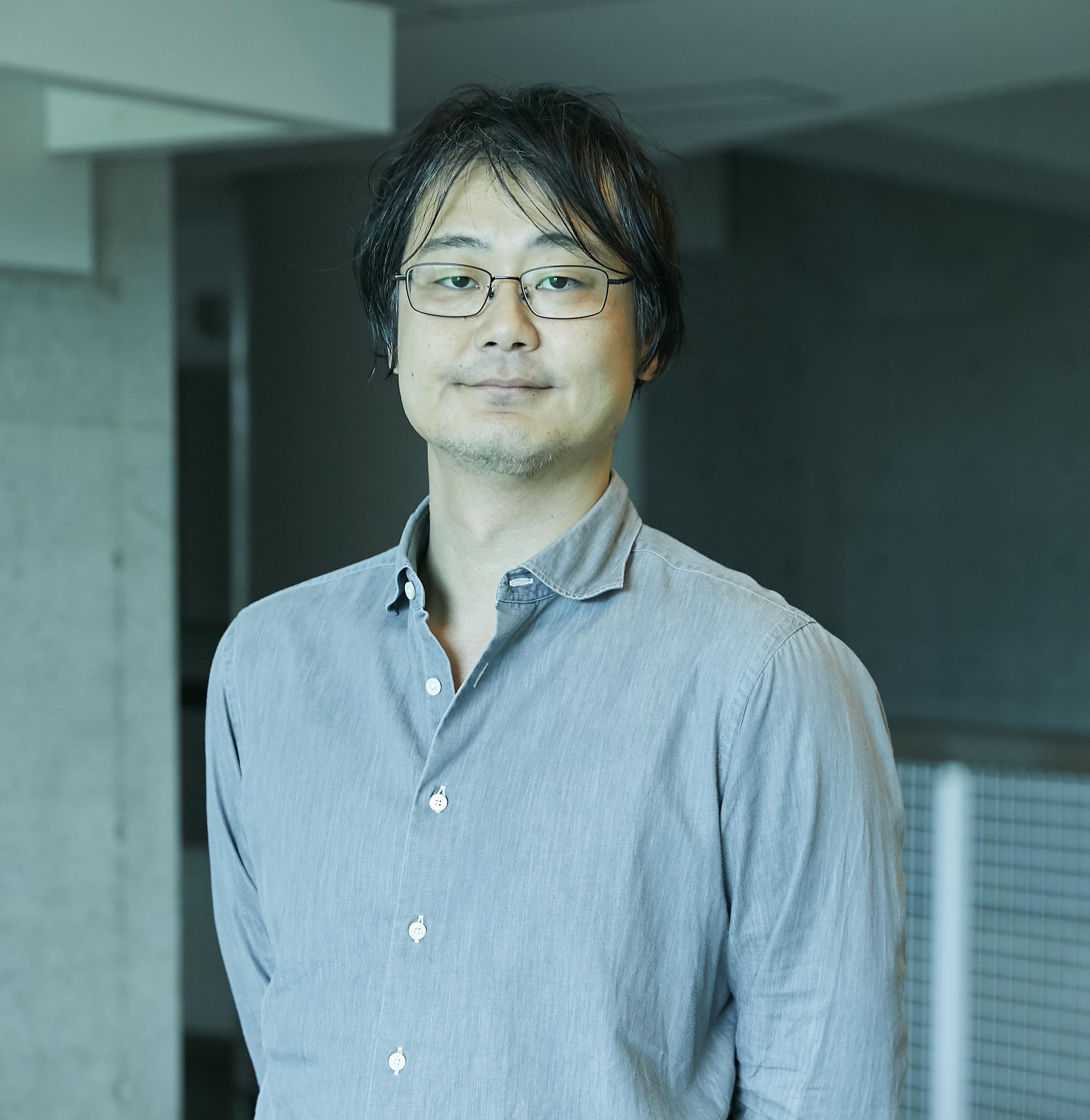
HAYASHI Shotaro
- Specialized field
Crystal Engineering, Polymer Science, Organic Synthesis, Organic Materials Chemistry
Macromolecules are used everywhere in our daily lives--in rubber and plastic products, paints, clothing, and numerous other applications. They have molecular weights of 10,000 or higher, and are also known as polymers, the generic term for extremely large molecules. Associate Professor Shotaro HAYASHI is striving to create "new materials beyond polymers" which have outstanding mechanical processability. He plans to achieve this through organic/polymer synthesis and molecular assembly chemistry focused on π-conjugated molecules, which are often used as organic electronics materials due to their unique electronic and optical properties.
Light-emission is an opportunity for application development
Molecular crystals have various functions, such as optical properties, electrical conductivity, and magnetism, and are a key material for realizing next-generation photonics and electronics. However, molecular crystals have a dense anisotropic structure where molecules are packed together, so although there is potential for higher performance, they lack flexibility, and are brittle and break easily. If we can freely design soft, pliant crystals, then perhaps new life can be brought to the materials field. With that idea in mind, Dr. Hayashi carried out crystal design based on molecule structure, using π-conjugated molecules (which have particularly high functionality among molecular crystals) to create "flexible molecular crystals" with density, anisotropy, and flexibility. In this process, he also discovered that these crystals have the property of emitting light and their light emission changes due to bending.
For Dr. Hayashi, these discoveries were truly a golden opportunity. He is currently focusing on applications in flexible optical waveguides and optical resonators, which exploit the elastic deformation and light-emitting characteristics of flexible molecular crystals.
Dramatic improvement in photon transport functionality of flexible molecular crystals
To realize nano-micro-small optical communication devices, it is crucial to develop "self-emitting" optical waveguides requiring no contact with the light source and no angle adjustment because they employ light emission by the waveguide itself. However, previous self-emitting types lacked flexibility and strength, and when there is overlap of the substance's light absorption band and light emission band, the device absorbs its own light emission, resulting in an issue of low phototransport efficiency.
Developing diverse types of optical resonators using organic crystals and polymer crystals
Light has the property that once emitted, it diffuses, and a key question for achieving free control of light is how to strongly confine it to a micro-region. Ultra-small "optical resonators," whose structure confines light for a certain time, are used in practice in various applications, like laser oscillation, as devices which save energy because signal processing is performed with the confined light. Dr. Hayashi and colleagues are also developing high-performance, ultra-small optical resonators by using flexible molecular crystals.
In principle, an optical resonator works like this: incident light is confined in a space, and the signal is amplified by sending it back and forth, or in a loop, inside the device so that a specific stationary wave is produced, and a distinctive type of light is emitted. There are various types of optical resonators, but the most typical is a Fabry-Pérot mode resonator which achieves resonation by passing light back and forth between two mirrors arranged parallel to each other. Molecular crystals made up of regular arrays of molecules can easily assume a parallel configuration. Thus, Dr. Hayashi produced various flexible molecular crystals, and measured changes in their fluorescence spectrum due to bending deformation. This resulted in discovery of patterns derived from the resonance mode, and a Fabry-Pérot mode resonator employing a flexible molecular crystal was successfully developed.
Aside from this, Dr. Hayashi is developing various types of optical resonators exploiting crystal flexibility, such as ring resonators where the confined light continues to loop while being fully reflected. This type is achieved by forming flexible molecular crystals into a ring shape.
Organic/inorganic hybrid spheres with light-emitting characteristics have also been created through the simple adjustment of applying light-emitting polymer solution to ultra-small silica spheres about 5μm in size. When light was shined onto these spheres and confined, and changes in the fluorescence spectrum were measured, it was discovered that sharp light emission bands periodically appeared, indicating optical resonance inside the sphere. This showed that these spheres behave as Whispering Gallery Mode (WGM) resonators, a type of ring resonator.
"WGM is a mode where light travels along circular walls. It's like if you whisper inside a large circular dome, the waves of sound are transmitted by the walls, and your voice can be heard by a person on the other side of the dome. The WGM mode works on the same principle as this phenomenon. Light travels around near the surface of the sphere, strongly resonating, so the oscillating light is sharpened, and this is expected to improve light output and light detection sensitivity."
Dr. Hayashi believes the scope of application will greatly expand when this light-emitting organic/inorganic hybrid sphere is coated on various substrates for greater surface area.
Aiming to establish a new academic domain beyond the boundaries of science
Resonators are also a key component of laser oscillators, and Dr. Hayashi wants to expand his R&D on optical resonators to lasers.
If it's possible to change the current mainstream, inorganic laser media to organic types, it will also be very advantageous in terms of both cost and energy.
"There is future potential, I feel, in using organic materials to develop super-energy-saving laser oscillators that collect large amounts of weak light and convert it into laser light. Organics have more diversity of structure than inorganics, but so far only single molecules have been used in applied science. In recent years, as the fusion of organic chemistry and physics has progressed, a foundation for exploiting that diversity has come together. Exploiting that foundation will greatly boost the potential for creating new materials."
Due to opportunities like this, Dr. Hayashi has recently participated in applied physics and laser conferences, and he is actively collaborating with other fields.
Even before that, in his laboratory, he designed and synthesized molecular structures starting from the functions he wanted them to manifest, devised design methods for crystal structures where many molecules are arranged in an orderly structure, and carried the development through to the end--not just evaluation of the characteristics which appeared, but also applications of the developed materials. Dr. Hayashi is committed to an interdisciplinary research style, and revolutionary research results have been consistently announced by his laboratory over the last few years. Many have attracted outside attention, being featured in academic journals and other media both inside and outside Japan. What drives this rapid progress?
"I do interdisciplinary research simply because I enjoy it. When I create a new substance, I don't stop there. I look at it from every angle, and persistently investigate its unknown points. That's what really motivates my research. Kochi University of Technology has an excellent culture that facilitates collaboration with other fields in research and interdisciplinary joint research. That sort of research environment is definitely a plus for me."
Dr. Hayashi's biggest goal, on which he's staked his research life, is to systematize paths from molecule design to applied development of devices, and establish a new academic domain that transcends the boundaries of materials science. Going forward, he will continue pushing toward its realization with original ideas and sound practical skills.
Date of posting: March, 2024/ Date of interview: October, 2023